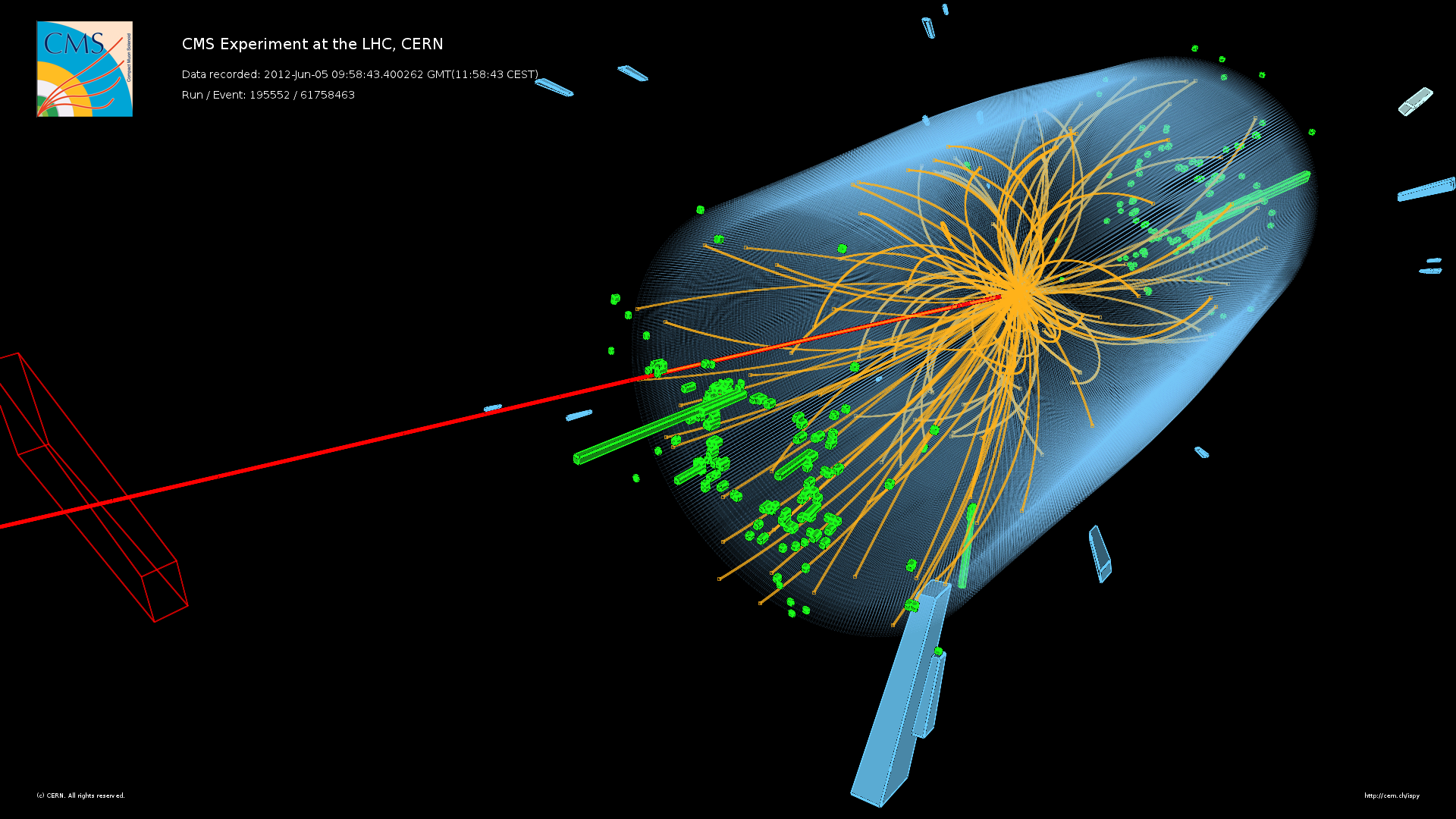
At a seminar held at CERN this morning, the CMS collaboration presented several measurements of the properties of the Higgs boson discovered in July 2012. CMS showed for the first time strong evidence for the decay of Higgs bosons into fermions, corroborating CMS results shown earlier this year. CMS physicists have now measured the decay of the Higgs to pairs of bottom (b) quarks and pairs of tau (τ) leptons with a combined significance of 4.0 σ[1] as compared to 3.4 σ previously. This significance means that the probability of a false positive, assuming no signal, is estimated to be only about one in 16,000.
The decay to fermions of the particle with a mass of around 125 GeV[2] discovered last year is an important confirmation that it behaves like the Standard Model (SM) Higgs boson. Since its discovery by CMS and ATLAS, both collaborations have studied its properties carefully, confirming, for example, that the particle is in fact a Higgs boson.
The Higgs, being an unstable particle, decays into pairs of lighter particles almost immediately after it is produced in proton collisions in the LHC. In general, particles can decay into various combinations of daughter particles. The Standard Model of particle physics gives very precise predictions for what the decay products are and how often they occur.
The Higgs boson has also been observed decaying into three types of gauge bosons: the Z, the W and the photon. The current evidence for these types of decays is extremely strong. For instance, CMS measures a significance of roughly 7 σ in the Z channel alone. The Standard Model also predicts decays to fermions –– namely quarks and leptons, which are the fundamental particles of matter. The fermionic decays into b quarks and τ leptons are particularly strong: they are the heaviest fermions that a Higgs with a mass of around 125 GeV would decay into and are consequently the most likely fermionic decays to occur. Indeed, the b-quark channel is the most common of all Higgs decays at this mass but background processes make it very difficult to extract a signal. On the other hand, decays to τ leptons, which can be thought of as heavier cousins of the electron, offer “cleaner” signals.
Using 4.9 fb−1 (inverse femtobarns[3]) of data collected at a collision energy of 7 TeV in 2011 and 19.7 fb−1 collected at 8 TeV in 2012, CMS has now completed refined searches for τ decays with several improvements over previous analyses and found an excess in this channel corresponding to significance of 3.4 σ. Together with earlier CMS searches for b decays that revealed a 2.1σ excess[4], excesses in the two channels have a combined significance of 4.0 σ (compared with an expected significance of 4.2 σ for a SM Higgs), indicating strong evidence for Higgs decaying to fermions. The observed rate of Higgs production with subsequent decay into b quarks or τ leptons divided by the expected rate for a SM Higgs gives a ratio (μ) of 0.90 ± 0.26, suggesting that the particle behaves like a SM Higgs.
Observing this decay further supports the conclusion that the Higgs boson discovered at the LHC is like the one predicted by the Standard Model but does not yet allow one to conclude that it is the same particle. There are models that predict multiple Higgs bosons of which one behaves just like a SM Higgs. CMS continues to look for additional Higgs bosons. Moreover, slight deviations from SM predictions in the properties of the discovered particle would also be clear indication of new physics beyond the Standard Model.
Discovering the boson was only the first step to further our understanding of our Universe. The precision measurements performed since then have taken many months and continue to shed light on the nature of the boson. The LHC, which is currently in its first Long Shutdown (LS1), will resume operations in 2015 with around twice the energy we’ve seen so far, providing several times more data for CMS physicists to pore over. The exciting journey has only begun.
The ATLAS Collaboration also presented their results for Higgs decays to fermions at a CERN-LHC seminar.
Resources
A collection of CMS Higgs resources can be found here: http://cmsexperiment.web.cern.ch/content/cms-higgs-resources
About CMS
More information: http://cern.ch/cms, or contact: cms-outreach@cern.ch.
CMS is one of two general-purpose experiments at the LHC that have been built to search for new physics. It is designed to detect a wide range of particles and phenomena produced in the LHC's high-energy proton-proton and heavy-ion collisions and will help to answer questions such as: "What is the Universe really made of and what forces act within it?" and "What gives everything mass?" It will also measure the properties of well-known particles with unprecedented precision and be on the lookout for completely new, unpredicted phenomena. Such research not only increases our understanding of the way the Universe works, but may eventually spark new technologies that change the world in which we live as has often been true in the past. The conceptual design of the CMS experiment dates back to 1992. The construction of the gigantic detector (15 m diameter by nearly 29 m long with a weight of 14000 tonnes) took 16 years of effort from one of the largest international scientific collaborations ever assembled: CMS has over 3000 physicists (including over 1500 students) plus around 800 engineers and technicians, from 182 institutions and research laboratories distributed in 42 countries.
Footnotes
[1] The standard deviation describes the spread of a set of measurements around the mean value. It can be used to quantify the level of disagreement of a set of data from a given hypothesis. Physicists express standard deviations in units called “sigma” (σ). The higher the number of sigma, the more incompatible the data are with the hypothesis. Typically, the more unexpected a discovery is, the greater the number of sigma physicists will require to be convinced.
[2] The electron volt (eV) is a unit of energy. A GeV is 1,000,000,000 eV; a TeV is 1000 GeV. In particle physics, where mass and energy are often interchanged, it is common to use eV/c2 as a unit of mass (from E = mc2, where c is the speed of light in vacuum). Even more common is to use a system of natural units with c set to 1 (hence, E = m), and use eV and GeV as units of mass.
[3] An inverse femtobarn at the LHC corresponds to about 100 trillion proton-proton collisions. Read more about this unit at http://news.stanford.edu/news/2004/july21/femtobarn-721.html
[4] The CMS paper Search for the standard model Higgs boson produced in association with a W or a Z boson and decaying to bottom quarks was recently accepted for publication in Physical Review D.
- Log in to post comments